High-T cuprates
c
Copper oxides (cuprates) with CuO2 sheets sandwiched by charge-reservoir spacer layers (see right figure) are known to host a plethora of quantum electronic phases. In the undoped 3d electronic configuration, cuprates are antiferromagnetic Mott insulators with a large charge gap (1-3 eV) and an exceptionally high Neél temperature (~1000 K). Upon doping of extra carriers, holes or electrons, the static antiferromagnetism (AFM) is rapidly suppressed and gives way to a “dome” of superconductivity (SC) with a maximal critical temperature Tc ~100 K on the hole-doped side and ~30 K on the electron-doped side. The mechanism behind the high-Tc cuprate superconductivity has been an active theme of research in condensed matter research since its discovery in 1986.
9
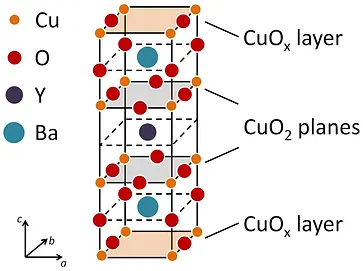
Crystal structure of YBa2Cu3O6+x, the first superconductor with a maximal Tc exceeding 77 K, the boiling point of liquid nitrogen. Figure reference: Sci. Rep. 4: 6250
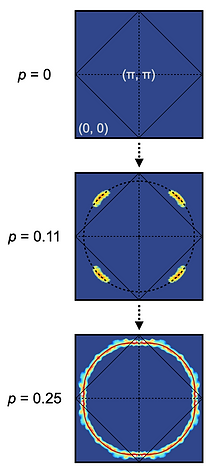
In addition to the AFM and SC phases, the cuprates host other intriguing electronic states of matter, including the pseudogap and charge-ordered state in the underdoped side (p < popt) and the strange metal phase in the optimal to overdoped side (p ≥ popt). The pseudogap (PG) is a peculiar state in which the electronic spectral weight is selectively suppressed along the
(π, 0) and (0, π) directions in the Brillouin zone, leaving behind broken ‘Fermi arcs’ in its non-superconducting normal state at T ≫ Tc. Intriguingly, the pseudogap share the same d-wave symmetry as the superconducting gap, leading to the belief that PG and SC in the cuprates are intrinsically linked. It is well established that the pseudogap opens at p ≤ p* ≈ 0.19, with recent experiments suggesting that p* is a quantum critical point, at which a quantum phase transition driven by quantum fluctuations occurs at zero temperature. However, this interpretation remains controversial as experimental evidence for a symmetry-breaking order parameter within the PG regime remains elusive.
Doping evolution of the Fermi surface of hole-doped cuprate superconductors. As holes are introduced into the half-filled CuO2 plane, the electronic ground state transitions from a Mott insulator (p = 0) to a paramagnetic metal (p = 0.25) through a 'pseudogap' state with discontinuous Fermi arcs. Figure reference: Nat. Phys. 4, 527 & New J. Phys. 9, 28
The strange-metal (SM) phase is another remarkable territory of the cuprate phase diagram. For ordinary metals, the resistivity (ρ) versus temperature (T) curve shows clear saturation at low temperatures (T → 0) and high temperatures (T → ∞). The saturation of resistivity reflects the diminished quasiparticle scattering against other bosonic modes at the temperature extremes. In the cuprates near the optimal doping, however, ρ(T) is linear-in-T from below Tc (when SC is suppressed, for example, by magnetic field) and up to the melting point (~1000 K), cruicially with the same slope (dρ/dT), suggesting a breakdown of the quasiparticle and the emergence of a new law governing the electronic transport. Unconventional transport behavior is also found in the magneto-resistance (MR) behavior, for which a linear-in-field MR is found at sufficiently high field strength. As the strange-metal phenomenology is ubiquitously found in unconventional superconductors, understanding strange metallicity in strongly correlated systems is currently a high priority in quantum materials research.
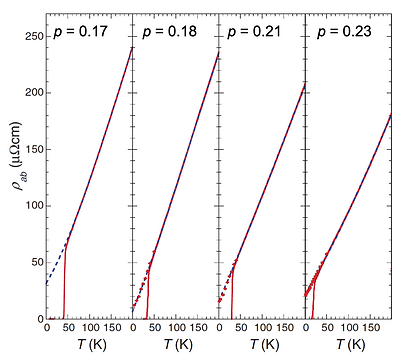
Resistivity in the strange-metal regime of La2-xSrxCuO4. A predominantly linear-in-T resistivity is found as T approaches zero, when superconductivity is suppressed by magnetic field. Figure reference: Science 323, 603
Further reading
1. B. Keimer et al., "From quantum matter to high-temperature superconductivity in copper oxides", Nature 518, 179 (2015)
2. C. W. Chu et al., "Hole-doped cuprate high temperature superconductors", Physica C 514, 290 (2015)
3. R. L. Greene et al., "The strange metal state of the electron doped cuprates", Annual Review of Condensed Matter Physics 11, 213 (2020)
4. P. W. Phillips et al., "Stranger than metals", Science 377, 169 (2022)